
GEOPHYSICS: Silent Slip
on the Cascadia Subduction InterfaceWayne
Thatcher*
Geodetic satellites of the Global Positioning
System (GPS) now permit continuous recording of surface motions
around earthquake faults and volcanoes with millimeter precision.
Data from distinct points on Earth's surface can be combined to
infer the locations of the sources of deformation at depth in the
crust. These data are radically altering our understanding of
earthquake processes, justifying ambitious new sensor arrays to
image active deformation sources in Earth's crust. In recent years,
large continuous GPS arrays have been deployed in Japan and southern
California, and smaller networks have been installed in other
seismically active regions. On page 1525
of this issue (1),
Dragert et al. provide a glimpse of the kinds of insights
we can expect from these arrays.
Dragert et al. use data from a 14-station continuous GPS
net located in southwestern British Columbia and northern Washington
to detect an episode of silent (aseismic) slip on a major fault that
surfaces offshore and dives eastward beneath the continent. This
fault defines the Cascadia subduction zone, which separates the
large, ~100-km-thick blocks of the Juan de Fuca and North American
plates (see the figure immediately below). Geological studies (2)
have shown that large earthquakes occur along the Cascadia plate
boundary roughly every 600 years. Modeling of geodetic data (3)
indicates that the shallow, upper ~20 km of the plate boundary fault
is currently "locked" (not slipping) because of frictional
resistance on the fault interface. Continued relentless motion of
the plates and aseismic slip increase stresses on the overlying
earthquake fault. These stresses will eventually be relieved by
abrupt shallow slip in a large earthquake.
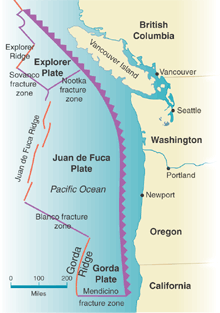 The Cascadia subduction
zone. Subdiction of the Juan de Fuca plate beneath the
North American plate results in the formation of the Cascade Range.
The Cascadia slip episode studied by Dragert et al. is
unique and important because it occurred beneath an apparently
completely locked plate boundary fault and was unrelated to any
local earthquake activity. Aseismic events reported elsewhere
occurred at the transition between locked and freely sliding fault
segments (4),
and many of them were triggered by transient adjustments after large
earthquakes (5,
6).
In contrast, the Cascadia event occurred on the downward extension
of the locked earthquake-generating fault, where aseismic slip or
distributed ductile shearing (7)
was expected to occur at a uniform speed. The physical processes
responsible for all such deformation instabilities are not much
studied or well understood. If similar episodes are identified
elsewhere, they will doubtless stimulate new work directed toward
understanding their mechanisms.
Aseismic events of the kind identified by Dragert et al.
have potentially important implications for earthquake occurrence.
Each aseismic slip episode perturbs the local stress field and may
bring a fault closer to failure. No single deformation episode is
necessarily an earthquake precursor, but if the locked fault is near
the end of its stress buildup cycle, an episodic slip event may be
sufficient to trigger a large earthquake (8).
The Cascadia slip episode, which took about 35 days, increased
stresses across the shallower, earthquake-generating part of the
plate boundary fault (white line segment in the figure immediatley
below). This stress increase associated with the event is very
small, equivalent to about half a year of steady stress buildup,
bringing the fault very slightly closer to failure. The maximum
change is about a factor of 10 smaller than the stress changes
caused by earthquake slip that have triggered subsequent earthquake
events (9).
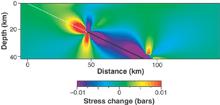 A small step toward
failure. Cross section showing elastic stress changes due
to silent slip as inferred by Dragert et al. on fault
segment shown in black. Positive stress changes (warm colors) bring
earthquake faults like that shown by the white line closer to
failure.
The Cascadia subduction zone last experienced a great earthquake
in 1700 (10)
and so may be only about halfway through its ~600-year earthquake
cycle. Therefore, even events substantially larger than the 1999
aseismic slip episode may not soon push this fault over the brink.
However, other regional faults may be closer to failure and such
episodic events could lead to large earthquakes. Continuous
monitoring of these faults, in Cascadia and elsewhere, is thus of
major importance.
Much remains to be learned about earthquake stress buildup. We do
not yet know whether aseismic episodes are rare or common, large or
small. For earthquakes, the numbers of events increases roughly
tenfold for each unit decrease in earthquake magnitude. For aseismic
events, we must determine the relation between frequency of
occurrence, slip, and slipped area to understand what causes them
and evaluate whether such episodes will trigger large earthquakes.
For the Cascadia episode, the ratio of fault slip to fault area was
quite small, about two orders of magnitude less than that typical
for earthquake slip. This ratio is proportional to the stress change
caused by the event, so the magnitude of the effects like those
shown in the figure is less important when the ratio is small.
Recent work (1,
4-6)
has revealed a rich spectrum of aseismic behavior in seismically
active regions but has also raised many new questions. Further
progress in understanding these processes depends on the deployment
of dense networks. Focused instrument clusters are needed to
spatially resolve buried sources of deformation, many of which could
be much smaller or more localized than that identified with the
relatively sparse array used by Dragert et al. These arrays
should also include ultrastable borehole strain meters, which are
increasingly more sensitive than continuous GPS for time intervals
of a month or less. Parallel developments are needed in refining
analysis and modeling techniques aimed at extracting the maximum
information from these large data sets (11).
Local prototype arrays of continuous GPS and borehole strain
meters have recently been installed by the U.S. Geological Survey at
Parkfield on the central San Andreas fault and at Long Valley
caldera, a region of volcanic unrest in eastern California.
Additional clusters in a range of geological environments will
ensure timely acquisition of the kinds of data needed to capitalize
on the capabilities of continuous GPS and borehole strain meter
technology and rapidly expand our understanding of how earthquakes
occur and why volcanoes erupt.
References and Notes
- H. Dragert, K. Wang, T. K. James, Science
292, 1525
(2001).
- B. F. Atwater et al., Earthquake Spectra
11, 1 (1996).
- P. Fluck, R. D. Hyndman, K. Wang, J. Geophys.
Res. 102, 20539 (1997) [ADS].
- A. T. Linde et al., Nature
383, 65 (1996) [GEOREF].
- K. Heki, S. Miyazaki, H. Tsuji, Nature
386, 595 (1997) [GEOREF].
- S. Ozawa, M. Murakami, T. Tada, J. Geophys. Res.
106, 787 (2001) [ADS].
- Aseismic slip occurs over a single discrete fault surface;
ductile shearing is distributed over a tabular volume of rock.
- W. Thatcher, Nature 229, 12 (1982).
- R. S. Stein, Nature 402, 605 (1999)
[ADS].
- K. Satake, K. Shimazaki, Y. Tsuji, K. Ueda, Nature
378, 246 (1996).
- P. Segall, M. Mathews, J. Geophys. Res.
102, 22391 (1997) [ADS].
The author is at the U.S. Geological Survey, Menlo Park, CA 94025,
USA. E-mail: thatcher@usgs.gov
Related articles in Science:
- A Silent Slip Event on the Deeper Cascadia Subduction
Interface
- Herb Dragert, Kelin Wang, and Thomas S. James
Science 2001
292: 1525-1528. (in Reports) [Abstract]
[Full
Text]
Volume 292, Number 5521, Issue of 25 May 2001, pp. 1495-1496.
Copyright © 2001 by The American Association for the
Advancement of Science. All rights reserved.
|